Review Article
Volume 2 Issue 4
Biodegradable polymers for sustainable environmental and economic development
Ibrahim MS,1 Sani N,2 Adamu M,2 Abubakar MK2
1Department of Chemistry, Sa’adatu Rimi College of Education, Kumbotso, Kano, Nigeria
2Department of Chemistry, Federal College of Education, Zaria, Nigeria.
Received: July 19, 2018 | Published: August 06, 2018
Correspondence:
Ibrahim Muhammad Shamsuddin, Department of Chemistry, School of Science Education, Sa’adatu Rimi College of Education, Kumbotso P.M.B 3218, Kano State, Nigeria, Email
Citation:
Ibrahim MS, Sani N, Adamu M, et al. Biodegradable polymers for sustainable environmental and economic development. MOJ Biorg Org Chem.
2018;2(4):192‒194. DOI: 10.15406/mojboc.2018.02.00080
Abstract
Bio-based polymers have become feasible alternatives to traditional petroleum-based plastics. In recent years, there has been a marked increase in interest in biodegradable materials for use in packaging, agriculture, medicine, and other areas. Polymers form the backbones of plastic materials, and are continually being employed in an expanding range of area. As a result, many researchers are investing time into modifying traditional materials to make them more user-friendly, and into designing novel polymer composites out of naturally occurring materials. A number of biological materials may be incorporated into biodegradable polymer materials, with the most common being starch and fiber extracted from various types of plants. The belief is that biodegradable polymer materials will reduce the need for synthetic polymer production (thus reducing pollution) at a low cost, thereby producing a positive effect both environmentally and economically. This paper is intended to provide a brief outline of work that is under way in the area of biodegradable polymer research and development.
Keywords: biopolymer, degradation, PHA, sustainability assessment
Introduction
The alarming awareness related to environmental and waste management issues around the universe, status and negative effects of fossil resources, are some of the reasons why biodegradable polymers and the need for their usage is increasingly promoted for sustainable development. Moreover, there is no sustainable economic development without a sustainable environment. Researchers universally have proven that fossil resources pose a serious threat to existence of humans, plants and animals by the generation of greenhouse gases and CFC’s. Critically observing the scenario, there is no need to exaggerate the importance of the safe and healthy environment to universal sustainable development. It is a prerequisite on which human and other living things existence lies. Biodegradable biopolymers from research results do not pose such a threat to the environment and its inhabitants compared to fossil based polymers, and therefore, there is need for biodegradable biopolymers universally. Abaza et al., cited in Pathak et al.1 opined that any global scheme of development created without taking the environment into account will actually be a house built on sand.
The Plastic industry in its initial stages promised to be a boon to mankind. Becoming a must and an instrument which is over used its impact on the environment and the society at large has become imperative. Recent research focus is on the production and qualification of Biofuels derived from plants, such as bioethanol which can be gotten from sugarcane and carbohydrates and biodiesel also from various plants, kitchen waste inclusive, plants are a prospective source of a much diverse range of useful chemicals and biomaterials. Biopolymers presently occupy a very small but emergent share of the polymer market. Some of these materials have distinctive properties that make them superior, user friendly, safer to the environment compared to synthetic polymers, especially in medicine, agriculture, engineering, textiles, where biocompatibility and biodegradability are strong assets. Thus, since fossils fuels also destroy agricultural farmlands via spills, reduction or total abandonment and embracing of biopolymers should be encouraged, taking careful consideration of the southern part of Nigeria where petroleum is being drilled, their land is not good at all for agriculture and thus, they have to depend on other parts of the country for a lot of agricultural produce, some animals inclusive because no available food for farming. Therefore to eliminate such environmental problems and to improve the effectiveness of biomaterials. Solaiman et al.,2 opined that to improve the effectiveness of new biopolymers and production technologies are required.
The cost of biopolymers needs to be looked into objectively and addressed, because as biopolymer is developing, more ideas about biopolymers and the need to embrace their usage is necessary and important, therefore, Economic concerns must be addressed, because the future of each biopolymer product is solely dependent on its cost competitiveness, and society’s ability to pay for it because most of the biopolymers are costly and since petroleum based polymers are cheaper, industries embark on their usage without considering the environmental factors rather the profit. In developed and developing countries, governments and NGO’S are introducing initiatives designed to promote, enlighten people and promote education by giving research grants, provide room for application and adequate development of biopolymers. Most countries all over the world and their policy makers support work in the area of biopolymer research, with government’s universally interested e.g. German government being particularly interested.3 This literature review provides information providing awareness and progress made available in the production, application and development of biodegradable polymer materials and some important information needed to be explored about biodegradable materials.
Historical outline
Biopolymers such as latex and cellulose have been used for production of rubber and plastics since the mid-1800s. PHA as a carbon and energy storage polymer, whereas PLA is produced from lactic acid that can be biologically derived from lactic acid fermentation. Application of PHA as a plastic began with work by the W.R. Grace Company in the 1950s, but most commercial development of bacterial PHA fermentations was performed by ICI during the 1960s and 1970s.4 The application of LCA to PHA production began with independent efforts at Dartmouth and Monsanto to evaluate, respectively, the environmental impacts of producing PHA by fermentation and in crop plants.5,6 These studies led to recognition of processing energy as the primary factor in the environmental impact of PHA production. Polylactic acid polymers were first investigated in the 1930s by Caruthers at DuPont. Many researchers investigated polylactic acid and polylactides over the next 50years. Cargill developed the technology for making lactides and purifying them with distillation while controlling the optical composition and purity of the lactides. This allowed Cargill to harness the polymer property variations that various lactide optical properties provide to polylactide polymers.
Degradation properties
Polymer degradation is a change in the properties-tensile strength, color, shape, etc-of a polymer or polymer based product under the influence of one or more environmental factors such as heat, light or chemicals. Degradation can also be induced deliberately to assist structure determination. Polymeric molecules are very large (on the molecular scale), and their unique and useful properties are mainly a result of their size.7 Any loss in chain length lowers tensile strength and is a primary cause of premature cracking (Figure 1). Surgeries are most common in India. Mostly metal plates are kept as the supporting material but now it is replaced by degradable polymer materials .The advantages of this are as follows:
- Do not require a second surgery for removal;
- Avoid stress shielding;
- Offer tremendous potential as the basis for controlled drug delivery;
- As the above figure explains the graph between mechanical strength and time (Figure 2)
Figure 1 Picture of raw Kaolin.
Figure 1 Picture of raw Kaolin.
Applications of biodegradable biopolymers
Biopolymers are applied in various fields such as medicine, research, agriculture among others because of their ability to degrade within a short lifespan compared to petroleum based polymers and thus such is presented in the diagram below (Figure 3).
Sustainability assessment of biobased polymers
Figure 1 Picture of raw Kaolin.
Life Cycle Assessment is a tool that can quantify the environmental impacts of biopolymers. However, the environmental impacts associated with the creation, use, and disposal of biopolymers remains unclear, since biopolymers can be made into a variety of products for a variety of uses, and ultimately are disposed of in many different ways. One role of LCA practitioners is to identify current production benchmarks and to analyze future scenarios to help guide the development of manufacturing, use, and disposal for sustainable products. The potential benefits of biopolymers in regards to GWP will not be realized until the material and energy demands from the farming and production processes are reduced. The use of REC’s in Vink’s 2003 and 2007 studies of PLA demonstrate the power of a low fossil fuel energy paradigm combined with carbon negative feedstocks.8,9 There is a great potential to sequester atmospheric carbon into everyday material. As noted by Gerngross et al. “any manufacturing process, not just those for plastics, would benefit from the use of renewable energy”.10 Life cycle analysis will continue to be a useful tool to identify more sustainable methods of production, use, and disposal of biobased products.11
The future prospects of biodegradable polymers
The impact of petroleum based polymers is becoming an issue to be looked into because of its negative impact to health, environment and economy since the price of the petroleum in the international market is getting low which provides low income to OPEC member states and this motivates researchers and industries into the field of biopolymer research. Thus, as a result of the unexpected outcomes of petroleum based products, research institutes and universities has plastic departments, e.g. Queen Mary University in London England has a plastics department which is actively working on biocomposite development.12 The more the biopolymer industry is well invested into universally and it grows, issues with production, price and quality has to be worked out since constant research on better alternatives are in progress, and also nanopolymer and biocomposites are still under research. There are some areas of concern that researchers are aware of, and are consequently focusing on. Multilayer films containing starch and/or natural fibers’ tend to have adhesion problems and thus natural fibers are also considered as alternatives for reinforcing matrices.13,14 Standards organizations such as the ASTM and ISO among others have published methods for material tests on biodegradable plastic materials in order to have environmentally friendly and cost effective biopolymer materials for sustainable development. Considering the nature, scope and characteristics of natural materials, there is a great need to give, consideration and due observation than the consideration and observation to be given to synthetic materials. Thus, biopolymer industry has a great positive future, which is driven mainly by the environmental and economic benefits of using natural resources for production of biomaterials.
Conclusion
Environmental sustainability is constantly increasing in importance to individuals, environment, and industries among others universally. Therefore, regulatory bodies such as ASTM among others should provide laid down policies governing the production and use of biodegradable polymers for sustainable environment and economic development.
Acknowledgements
None
Conflict of interest
Authors declare that there is no conflict of interest.
References
-
Swati P, Sneha CLR, Mathew BB. Bioplastics: Its Timeline Based Scenario & Challenges. Journal of Polymer and Biopolymer Physics Chemistry. 2014;2(4):84‒90.
- Solaiman DK, Ashby RD, Foglia TA, et al. Conversion of agricultural feedstock and coproducts into poly (hydroxyalkanoates). Appl Microbiol Biotechnol. 2006;71(6):783‒789.
- Simona J, Müllera HP, Kocha R. Thermoplastic and biodegradable polymers of cellulose. Polymer Degradation and Stability. 1998;59(1-3):107‒115.
- Asrar J, Gruys KJ. Biodegradable polymer (BiopolH). In Biopolymers–(Polyesters III–Applications and Commercial Products). In: Doi Y, Steinbuchel A, editors. Weinheim: Wiley-VCH; 2002. p. 53–90.
-
Gerngross TU, Slater S. How Green are Green Plastics? Sci Am. 2000;283(2):37‒41.
- Kurdikar D, Paster M, Gruys KJ, et al. Greenhouse gas profile of a plastic derived from a genetically modified plant. Journal of Industrial Ecology. 2001;4(3):107‒122.
- Blanco A. Just add water. Plastics Engineering. 2002;58(10):6.
-
Vink ETH, Glassner DA, Kolstad JJ, et al. The eco-profiles for current and near-future NatureWorks® polylactide (PLA) production. Industrial Biotechnology. 2007;3(1):58e81.
- Vink ETH, Rábago KR, Glassner DA, et al. Applications of life cycle assessment to NatureWorksTM polylactide (PLA) production. Polymer Degradation and Stability. 2003;80(3):403‒419.
- Gruber P, O’Brien M. Polylactides “Nature Works(TM) PLA”. In: Steinbuchel A, editor. Biopolymers. Weinheim: Wiley-VCH; 2002. p. 235‒239.
- Hottle TA, Melissa MB, Amy EL. Sustainability assessments of bio-based polymers. Polymer Degradation and Stability. 2013;98(9):1898‒1907.
- Hogg P. Plastics, rubber, and composites at Queen Mary. Plastics, Rubber and Composites. 2001;30(5):193‒194.
-
Frisoni G, Baiardo M, Scandola M, et al. Natural cellulose fibers: Heterogeneous acetylation kinetics and biodegradation behavior. Biomacromolecules. 2001;2(2):476‒482.
- Martin O, Schwach E, Averous L, et al. Properties of biodegradable multilayer films based on plasticized wheat starch. Starch. 2001;53(8):372‒380.
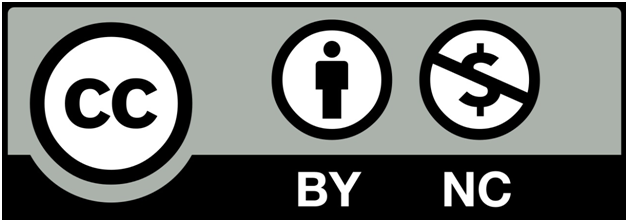
© 2018 Ibrahim et al. This is an open access article distributed under the terms of the Creative Commons Attribution License
, which
permits unrestricted use, distribution, and build upon your work non-commercially.
|
|